Wednesday, December 20, 2006
Wednesday, September 13, 2006
story on the TDU by Mr. Alan Dean
On Friday, September 8, 2006 I took part in a scientific study which enablesblind persons to be able to visualize objects by stimulation of theirtongue. After being introduced to theprogram , I was seated facing a wall, 3 feet in front of me. I was allowedto feel the shape of what turned out to be a very large letter E. The aparatus, described in the article below was set in place. After a fewminutes of adjusting my head up and down and from left to right, incarefully contrived movements, I was able to visualize the letter EThis wasexhilirating and I could hear my heart beating quite loudly. This figure wasthen turned in different directions and I had to describe what I was able tovisualize. As this part of the study continued, the size of the itemswere reduced until such time I was not able to discern the shape within thetimeframe.This was the first time I could visit the world I thought haddisappeared when I lost my sight. The rest of the study is adequatelydescribed in the transcript of the segment of the Television program airedby the ABC Networkacross North America.I cannot describe the wonderful feeling I experienced yesterday. I wasoverwrought with emotion and excitement and returned home, totally exhaustedbut overjoyed that whatever the outcome will be, in my lifetime or not, thatI have made a contribution to science and with the hope that many personswill be the beneficiaries of the contribution made by the persons who haveparticipated in this study. Mike Ciarciello is not only a musician but isalso an accomplished specialist teaching blind persons how to use a computer...
Alan Dean.
Friday, September 08, 2006
ABC prime time

: http://abcnews.go.com/Primetime/story?id=2401551&page=1.
September 9, 2006
Transcript of program follows:September 6, 2006
- - Mike Ciarciello has been blind since birth but saysthat in his dreams he can actually see."I have had dreams where I have been flying, you know, like in the air. I amnot even bumping into any obstacles whatsoever. I am actually free, in mydreams,"he said.His dreams are closer to reality than you might imagine. He is about toparticipate in an experiment in which he will "see" by using his tongue.At the University of Montreal, researcher Daniel Chabat prepared Ciarcielloto walk for the first time through an obstacle course without his cane.Chabatbegan by mounting a small camera on Ciarciello's forehead. The camera sendselectrical impulses about what it sees to a small grid placed on his tongue."It's a concept in which you replace a sense that was lost by another onethat is there," said Maurice Ptito, the neuropsychologist supervising thestudy."They sense the world through their tongue, and that gives them the feelingof seeing. You don't see with your eyes. You see with your brain."When ABC News correspondent Bob Brown tried The BrainPort vision device inan informal experiment, his challenge was to identify black shapes placed ona wall in front of him. As the camera scanned the shapes Brown described thefeeling on his tongue as a tingling sensation."It's a pulsing sensation that imprints in a crude way the shape of theobject," he said. "The closer I move to the object, the more the feelingintensifies."The tongue is used as the source of input because it is the first organ thatwe use, Ptito said."We've been using the tongue since we were born," he said. "It's easilyaccessible; it's a wet milieu, so it's a nice conductor. So it's a reallyfine tunedmachine, so to speak."Once Ciarciello had the camera mounted and connected to the grid on histongue, he was ready to head into what for him was completely unchartedterritory:the obstacle course."I hope it's going to be a great experience in the sense that I'm able toactually walk around an object without bumping into it and at my own will,"Ciarciellosaid.As he walked through the obstacle course for the first time, he bumped intoobjects. It takes training to learn to interpret the signals on the tongue,to sense the distance of objects and whether they're on the floor or infront of him, Ptito said."It took some getting used to, because I had to basically look up and down,left and right," Ciarciello said. "I am not used to doing that in aneverydayworld-type situation."But after just two hours of training he was walking through the maze,hitting fewer and fewer obstacles. For the first time, Ciarciello was ableto senseobjects in the distance, too far away to touch. His tongue, in a sense, wassubstituting for his sight."They believe that they can see. In the sense that they appreciate thevisual world, they can see things moving around, they can see things comingto them,"Ptito said.It may be more than just a belief, because when researchers scan the brainsof sightless people who have used the device the scan shows activity in boththe visual and motion areas of the brain, showing that one sense is beingsubstituted for another.The BrainPort" vision device was developed by Dr. Paul Bach-y-Rita of theUniversity of Wisconsin.
Copyright © 2006 ABC News Internet Ventures
Tuesday, August 15, 2006
Radio-Canada, Découverte

- Un œil sur la langue -
Rendre la vue aux aveugles est un vieux rêve de la science. On a déjà essayé en implantant directement une puce reliée à une caméra à même le cortex visuel, une opération risquée. On essaie maintenant d’utiliser un stimulateur lingual. Le neuropsychologue Maurice Ptito, de l’École d’optométrie de l’Université de Montréal, utilise une languette munie d’un processeur de 144 pixels. Il l’installe dans la bouche d’un aveugle, contre le palais. Avec la langue, l’aveugle perçoit les impulsions électriques qu’envoie une caméra et il essaie de distinguer les formes. La langue a plusieurs avantages. Elle baigne dans un milieu aqueux qui conduit bien l’électricité. De plus, c’est un organe très sensible qui apporte énormément de renseignements au cerveau.
Journaliste: Claude D’Astous Réalisateur: Pierre Tonietto
video:
http://www.radio-canada.ca/actualite/v2/decouverte/niveau2_8722.shtml
Tuesday, July 25, 2006
Eighth Edition of the Vision Network Prize - Huitième concours du prix réseau vision
It is a great pleasure for me to announce that my article on the development of the commissure of teh superior colliculus won me the 8th student vision network prize for the best student article. Please follow this link to the vision network website where you can download a copy of my article: http://reseauvision.ca/.
Daniel
Tuesday, July 04, 2006
Substitution sensorielle et plasticité intermodale
Université de Montréal
Substitution sensorielle et plasticité intermodale
par
Daniel-Robert Chebat
Texte de synthèse
présenté à la faculté des études supérieures
en vue de l’obtention du doctorat
sous la direction de
Maurice Ptito, Ph.D, M.D.
© 2006, droits reservés
Introduction
"L’activité principale des cerveaux est de créer des changements en eux mêmes"Marvin L. Minsky (dans Society of the Mind, 1986)
Le cerveau possède une étonnante capacité à réorganiser ses voies nerveuses selon l’expérience sensorielle qui lui est disponible. Cette capacité de changer en fonction de l’expérience sensorielle est ce que l’on nomme la plasticité du cerveau. L’activité module le développement du cerveau, mais le transforme aussi dans l’âge adulte. Il n’y a pas si longtemps, nous pensions que la plasticité du cerveau était confinée à une étape précise du développement et, qu’une fois les connections neuronales bien en place, le cerveau devenait figé. Cette conception de la neuroplasticité a beaucoup évolué durant les vingt dernières années. Nous savons aujourd’hui que le cerveau retient beaucoup de ses capacités plastiques tout au long de son existence. C’est grâce en partie à cette neuroplasticité que nous apprenons et que nos expériences sont transformées en mémoires (Kandel, 2001). Nos expériences semblent moduler la matière même qui nous permet de penser et de ressentir. Par exemple, le système visuel est modifié en fonction de l’intrant visuel (Wiesel & Hubel, 1965). L’environnement possède une influence immense sur le développement du cerveau : notamment, les animaux élevés dans des conditions enrichies présentent des changements dans l’épaisseur de leurs cortex et un agrandissement de l’espace dendritique pour les synapses en comparaison à des animaux élevés dans des conditions normales (Rampon & Tsien, 2000). Dans certains cas de lésions, cette plasticité a pour avantage adaptatif de sauvegarder certaines fonctions du cerveau qui seraient autrement perdues. Par exemple, des lésions précises de certaines zones visuelles peuvent entraîner des reconnexions anormales rétiniennes vers le cortex auditif chez le hamster (Schneider, 1973; Ptito, et al., 2001; Frost, et al., 2000), et le furet (Sur, et al., 1988). Dans le cas de la cécité, la plasticité permet d’augmenter d’autres fonctions, par exemple l’ouïe ou la somesthésie pour compenser l’absence d’un sens. Il existe certaines preuves chez l’humain (Sadato, et al., 2004) et chez l’animal (pour une revue voir : (Feldman & Brecht, 2005) que cette plasticité pourrait modifier le cerveau même à l’âge adulte. Ceci suggère que non seulement les personnes atteintes de cécité congénitale, mais aussi celles touchées de cécité tardive pourraient bénéficier d’appareils de substitution sensorielle par le biais de mécanismes plastiques.
L’ambition de pouvoir remplacer un sens par un autre fut d’abord celle de Louis Braille, suivi de Paul Bach-y-Rita et, aujourd’hui, nous héritons de l’espoir de pouvoir procurer un substitut acceptable aux personnes atteintes de cécité grâce à cette capacité de réorganisation du cerveau. L’objectif de ce texte de synthèse est de faire la revue de la littérature sur les thèmes de substitution sensorielle et de neuroplasticité dans le cadre des applications pour un système de substitution sensorielle chez les aveugles, sujets essentiels à mon étude sur les aspects neuro-cognitifs du processus de la navigation chez l’aveugle-né. Nous parlerons d’abord de la plasticité au niveau cellulaire et du développement du système visuel; nous aborderons ensuite les changements structuraux qui découlent de la cascade d’événements biochimiques qui sont à la base de la plasticité intermodale et de la substitution sensorielle chez l’animal, mais aussi chez l’humain.
1. Spécialisation corticale
Dans un premier temps, il conviendra de décrire ce qu’est la spécialisation corticale et de revenir sur les études menées sur le sujet ayant permis la découverte de la spécialisation corticale.
Le premier à avancer la thèse selon laquelle il existe une spécialisation des aires corticales était Francis Gall, selon lequel on pouvait prédire le comportement et la personnalité d’un homme en étudiant la forme de son crâne. Cette science se nomme la phrénologie.
La base scientifique de cette idée s’est révélée être fausse; toutefois, l’hypothèse principale, c'est-à-dire la spécialisation corticale, était correcte. L’étude du cerveau des soldats qui revenaient blessés des Première et Deuxième guerres mondiales apprit beaucoup aux chercheurs désireux d’étudier la spécialisation des aires corticales. Ces blessés revenaient souvent avec des éclats d’obus plantés à des endroits précis de leurs cerveaux. Très vite, on se rendit compte que, selon l’emplacement de la lésion, les troubles cognitifs étaient semblables ou dissemblables. On découvrit de cette façon l’aire de Broca et de Wernicke, c’est-à-dire les aires destinées au langage. On a pu faire le rapprochement avec des études de cas datant du début du siècle, dont le cas de Phineas Gage, cet homme dont la partie frontale du cerveau fut transpercée de part et d’autre par une barre de fer.
Suite à l’accident, la personnalité de cet individu fut brusquement transformée : il passa d’un tempérament calme et réservé à un comportement brutal et agressif. L’analyse post-mortem de son cerveau démontra que la lésion se situait dans le cortex frontal (Steegmann, 1962; Macmillan, 1986; Yorks, 2005). Or, nous savons aujourd’hui que le cortex frontal assure l’inhibition et la gestion des émotions (Wagar & Thagard, 2004). Ces révélations donnèrent naissance aux techniques de lobotomie pratiquées, entre autres, par le Dr. W. Penfield. Le Dr. Penfield fut aussi le pionnier des opérations d’hémisphérectomie pour traiter les cas graves d’épilepsie. Durant ses opérations pionnières, le Dr. Penfield identifia, à l’aide de l’utilisation de méthodes électro-physiologiques, différentes aires corticales dont le cortex temporal (Penfield, 1956-1957; Penfield, 1957b; Penfield, 1957a) et le cortex visuel (Penfield & Milner, 1958; Penfield, 1958), et le rôle de l’hippocampe dans la mémoire (Penfield & Milner, 1958). Toutes ces études démontrent que le cerveau possède des zones qui sont spécialisées dans certaines fonctions.
1.1. Le rôle de la plasticité dans le développement et la spécialisation corticale
Le développement du cerveau dépend de l’interaction entre notre prédisposition génétique et l’environnement (pour une revue voir : Ptito & Desgent 2005). L’interaction de ces deux facteurs durant le développement changera non seulement les connexions et l’organisation du cerveau, mais aussi la fonction des différentes aires corticales. Dans cette section, nous traiterons de la plasticité du cerveau au niveau cellulaire durant le développement normal du système visuel.
Le développement cortical comprend la formation de plusieurs aires capables de traiter différentes sortes d’informations de façon unique. Chaque zone est caractérisée par des intrants différents, par des réseaux de traitement intrinsèque et par des projections spécifiques. Durant le développement, la spécialisation corticale est dépendante de facteurs internes (génétiques et moléculaires) et de facteurs externes (expérience sensorielle provenant de l’environnement). Les facteurs internes génétiques prédisposent la segmentation grossière des aires corticales, mais c’est grâce à l’expérience sensorielle que ces réseaux sont maintenus en place (pour une revue voir Ptito & Desgent, 2006). L’apport développemental de la plasticité est de permettre au cortex de s’adapter en fonction des intrants sensoriels. La plasticité du système visuel a été démontrée chez plusieurs espèces et de diverses façons. Le hamster et le furet sont d’excellents modèles pour étudier la plasticité en raison de leur état prématuré à la naissance, permettant ainsi de faire des chirurgies post-natales à un stade de développement très précoce sans avoir recours à des embryons. Ces études permettent de constater les conséquences plastiques du cerveau suite à différentes formes d’atteintes, soit la cécité ou la destruction d’une aire corticale, ou même suite à l’ajout d’un troisième œil. La cascade d’événements biochimiques qui mènent éventuellement à des changements structuraux dépend de l’expérience visuelle pour un développement intermodal. L’intermodalité signifie la capacité d’une aire corticale de prendre en charge certaines fonctions d’une autre aire corticale par des mécanismes de plasticité. La section suivante décrira le rôle de la plasticité dans le développement normal et plus particulièrement dans la spécialisation du cortex visuel.
1.2. Développement du système visuel et plasticité
La différenciation initiale du cortex se fait par le biais d’indices moléculaires qui sont indépendants de l’expérience (Rubenstein et al., 1999). Dans le cortex visuel, par exemple, plusieurs aspects des connections thalamo-corticales sont mis en place avant le début de l’activité des yeux (Crowley & Katz, 2000; Sur & Leamey, 2001). Bien que des facteurs intrinsèques délimitent grossièrement les zones corticales, les afférents thalamiques peuvent plus tard influencer la grosseur et même l’identité de certaines zones en fonction de l’expérience (Sur & Leamey, 2001). Les influences sensorielles agissent donc sur la morphologie du cerveau par l’intermédiaire des afférences thalamiques. Le thalamus serait donc un important centre de relais entre la rétine et le cortex visuel.
Dans le développement normal d’un individu, l’expérience visuelle joue un rôle important dans le modelage des connections, et du cortex en général. Ceci est mis en évidence par les études de privation sensorielle où l’on peut voir les conséquences anatomiques de l’absence de vision. Les facteurs génétiques mettent en place l’anatomie grossière d’une zone, et c’est avec l’expérience visuelle que ces connections sont affinées. Par exemple, durant le développement de la commissure du collicule supérieur (CCS), existent des projections exubérantes dans les couches superficielles du collicule supérieur (CS), et ces projections sont éliminées juste après l’ouverture des yeux (Chebat, Boire, & Ptito, 2006)a. Si l’animal est privé d’expérience visuelle durant son développement, ces projections exubérantes dans les couches superficielles du CS ne sont pas éliminées car on observe une expansion de la CCS jusque dans les couches superficielles (Rhoades & Fish, 1982). Le corps calleux (CC) emploie aussi un mode de développement régressif qui est caractérisé par l’élimination de projections exubérantes en fonction de l’expérience visuelle (Innocenti, 1978; Innocenti & Frost, 1980; Olavarria & Hiroi, 2003). Le développement de ces deux commissures est un exemple de l’interaction entre les facteurs génétiques et l’expérience visuelle pour la formation de connexions inter-hémisphériques : les facteurs génétiques génèrent la possibilité de modifications par un effet d’exubérance; c’est ensuite à l’expérience visuelle de moduler ces connections. L’interaction entre l’environnement et la génétique opère de façon semblable pour la spécialisation corticale.
La spécialisation corticale commence tôt dans la vie embryonnaire avec la transcription de facteurs génétiques. Les patrons d’expressions qui en découlent sont responsables de la formation de voies corticales et de la mise en place des connections propres à chaque zone dont, par exemple, la connectivité entre le thalamus visuel et le cortex visuel (Sur & Rubenstein, 2005). Le cortex visuel est une structure hautement organisée; les cellules qui ont des réponses semblables sont groupées ensemble durant le développement pour former des représentations du champ visuel telles que les colonnes de dominance oculaire, la préférence à l’orientation et les cartes rétinotopiques (Sincich & Horton, 2005; Yu, et al., 2005). Tôt dans le développement, l’activité interne génère de l’activité spontanée qui façonne les circuits neuronaux en fonction d’un estimé génétique de la configuration requise pour la fonction et la survie.
Les colonnes de dominance oculaire dans V1 sont formées par la ségrégation d’intrants des deux yeux, relayée à travers les couches du corps genouillé latéral (LGN). Ces changements se font indépendamment de l’expérience visuelle puisqu’ils se produisent avant même l’ouverture des yeux. L’activité spontanée de la rétine conduit ces changements initiaux. Le maintient de ces colonnes dépend par contre de l’expérience visuelle. Sans l’expérience visuelle d’un œil, celui-ci perdra l’espace cortical qui lui a été alloué au profit de l’œil non-privé d’expérience visuelle.
Avec la maturation du cerveau, les premiers changements induits par l’expérience visuelle sur les circuits neuronaux sont sans doute des changements d’ordre d’efficacité des connectivités synaptiques. L’efficacité synaptique dépend de deux mécanismes : la potentialisation à long terme (PLT) et la dépression à long terme (DLT). La cellule présynaptique utilise ces mécanismes pour emmagasiner de l’information sur l’historique de l’activité sous la forme de Ca2+ dans ses terminaux. Cette formule est l’application du principe de Hebb (1949) : quand une cellule stimule une autre cellule, certains changements métaboliques se produisent dans l’une ou les deux cellules et augmentent l’efficacité des connections entre ces deux cellules. Le stockage de l’information biochimique dans la cellule après une brève période d’activité entraîne le renforcement de la connectivité synaptique pendant plusieurs minutes (Berardi, Pizzorusso, Ratto, & Maffei, 2003). Si au contraire un réseau cellulaire reste inactif, les mécanismes de DLT feront en sorte que ces réseaux seront moins renforcés et les cellules inactives mourront.
L’expérience visuelle module la différentiation corticale en régulant le taux d’éphrines des afférences thalamiques au cortex visuel (Sur & Leamey, 2001). Les éphrines sont des molécules qui négocient le contact entre les cellules, et sont impliquées principalement dans la genèse et la stabilisation des organisations cellulaires. Elles accomplissent cette tâche en contrôlant les mécanismes d’adhésion et de répulsion des cellules. La PLT et la DLT sont intimement liées aux récepteurs N-d-méthylaspartate (NMDA). L’énucléation binoculaire induit la réduction des apports thalamiques au cortex visuel, ce qui entraîne la création d’un cortex visuel « hybride » (Rakic, et al., 1991; Dehay, et al., 1996). Parallèlement, l’ablation complète du thalamus entraîne une réduction de la taille des zones corticales et de l’épaisseur du cortex (Miller, et al., 1991; Windrem & Finlay, 1991). Les études animales mettent en évidence l’apport immense qu’a la vision sur le système visuel grâce à la plasticité.
2. Études animales
2.1. Études sur la privation sensorielle et du strabismus
Depuis les études de Hubel et Wiesel en 1965, le modèle de la privation sensorielle et, plus précisément, de l’énucléation a été énormément étudié pour comprendre le développement du système visuel. Les études de privation sensorielle ont pour but de mieux comprendre le développement du système visuel, mais surtout l’apport de l’expérience visuelle dans le développement du cerveau. Les études de privation sensorielle comprennent les études d’énucléations uni ou bilatérales, les études d’élevage en noirceur, et la suture des paupières. Cette section décrira brièvement les études sur la privation sensorielle et leur impact sur notre compréhension du développement cérébral et de la plasticité qui en est le moteur principal.
Nous savons que si l’on enlève l’apport de l’un des yeux, nous changerons aussi les colonnes de dominance oculaire (Wiesel & Hubel, 1965), mais ces changements ne sont pas immédiats (Crowley & Katz, 1999). Ceci implique que ces projections sont mises en place par des facteurs internes, indépendants de l’expérience visuelle, mais que l’expérience visuelle est requise pour les garder en place. La plasticité de colonnes de dominance oculaire reflète l’interaction compétitive entre les deux yeux pour la conquête de territoire synaptique. L’énucléation favorise la formation d’espace synaptique pour les colonnes de dominance oculaire de l’œil sain au dépend de l’œil énucléé. De la même façon, le strabisme favorise l’espace cortical dédié à l’œil sain, au dépend de l’œil strabique. Le strabisme congénital engendre une réduction de l’acuité visuelle de l’œil dévié, et une diminution de sa capacité à contacter les neurones visuels corticaux (Ptito et al., 1995). Des neurones du cortex visuel primaire peuvent être dirigés par l’œil dévié, mais les cartes rétinotopiques reflèteront l’anomalie visuelle par un positionnement anormal des champs récepteurs de ces neurones.
Le cortex visuel du chat contient des cellules qui répondent de façon préférentielle à certaines orientations. L’élevage de chatons dans un environnement constitué exclusivement de barres orientées dans une certaine direction, restreint la vision des formes et change l’orientation préférée des cellules. On ne trouve plus de cellules pouvant répondre aux orientations absentes de l’environnement des chats, et on note une expansion des colonnes d’orientations dédiées aux orientations présentes dans ce même environnement (Sengpiel, et al., 1999). Ceci indique que l’expérience visuelle a un rôle instructif dans le développement des cartes d’orientation et la sélectivité à l’orientation.
Si l’absence de certaines orientations dans l’environnement d’un animal a un impact sur le développement de son cerveau, l’absence d’un sens sur le cerveau durant le développement entraîne une cascade d’évènements biochimiques qui peuvent aller jusqu’à changer l’identité d’une aire. Dans ce cas, l’aire qui est d’habitude dédiée à ce sens qui fait défaut se dévoue aux autres sens demeurés. Les neurones de l’aire visuelle du chat (AEV) sont activés par la stimulation auditive et tactile quand on prive ces animaux d’expérience visuelle dès la naissance (Rauschecker & Korte, 1993). En d’autres mots, les aires auditives et somatosensorielles avoisinantes se sont étendues dans l’aire visuelle. Pour compenser l’absence de vision, l’AEV de ces animaux aveugles possède des propriétés du cortex auditif (AEA), en plus du fait que les neurones auditifs de ces mêmes animaux ont une meilleure calibration pour la localisation d’un son dans l’espace que leurs congénères voyants (Korte & Rauschecker, 1993). Du point de vue comportemental, il semblerait que les chats aveugles parviennent mieux à localiser la source d’un son que les chats voyants (Rauschecker & Kniepert, 1994). Chez le rat énucléé, le cortex visuel prend également en charge des fonctions auditives et somatosensorielles (Toldi, et al., 1994)
Inversement, on peut aussi transformer le cortex auditif pour qu’il acquière des propriétés visuelles. Le cortex auditif du chat sourd peut ainsi s’approprier des fonctions visuelles (Rebillard, et al., 1977). Le rat-taupe est un animal aveugle qui dépend de l’information tactile et auditive pour ses déplacements sous terrains. La stimulation auditive peut activer des cellules du cortex visuel de cet animal aveugle-né (Bronchti et al., 2002). Ces résultats confirment que l’expérience et les afférences sensorielles ont un rôle important dans la spécification du rôle et de l’architecture des aires corticales. De la même façon que les yeux se font compétition durant le développement pour l’espace cortical, les aires corticales définies par les indices moléculaires et l’activité spontanée doivent se combattre pour maintenir l’espace cortical alloué génétiquement (Blakemore, et al., 1976; Guillery & Stelzner, 1970). C.est pour cette raison que l’on qualifie la relation entre les aires de compétitive. Si une aire est privée de son intrant sensoriel, elle cède l’espace à une autre fonction dont l’intrant sensoriel est intact. Cet espace cortical changera d’identité par le biais de mécanismes de plasticité.
2.2. Études additives
Une autre façon d’étudier les mécanismes de plasticité est d’ajouter un nouvel intrant sensoriel au cerveau. Par exemple, les études de greffes corticales démontrent la grande plasticité du cerveau. Concrètement, on retire une aire corticale à provient un animal et on la greffe au cortex d’un autre animal. La greffe d’un cortex occipital très immature sur une zone pariétale du cortex peut entraîner des changements profonds dans cette aire greffée. La zone greffée adopte des caractéristiques de sa zone hôte plutôt que sa zone d’origine; le cortex occipital développe des projections en tonneau. Ces projections sont propres au cortex somatosensoriel (Toldi, et al, 1994) et, pourtant, elles apparaissent dans le cortex visuel greffé. Les projections en tonneau sont des formations de cellules présentes dans la couche IV du cortex somatosensoriel du rat qui traite l’information d’une seule vibrisse de cet animal.
Une autre façon d’ajouter un intrant au cerveau est de greffer un nouvel organe périphérique et d’étudier les changements au niveau central. Il est possible à l’aide de méthodes chirurgicales délicates de greffer un troisième œil au cortex frontal de la grenouille.
L’apport de ce nouvel œil forme des terminaisons stéréotypées et spécifiques à cet œil, semblables aux colonnes de dominance oculaire que l’on trouve chez l’animal normal (Constantine-Paton & Law, 1978). La grenouille a donc développé des colonnes de dominance oculaire desservant l’œil transplanté (Law & Constantine-Paton, 1981). Si l’on injecte de la proline (un traceur antérograde) dans l’œil transplanté, on peut voir la partie du lobe tectal visuel qui a absorbé ce traceur. Ceci indique qu’il existe des connexions entre l’œil transplanté et ces colonnes de dominance oculaire (Law & Constantine-Paton, 1980).
Pour que ces colonnes de dominance oculaire se forment dans le lobe tectal, il est nécessaire que l’œil soit fonctionnel. En effet, l’activité de ce dernier est indispensable au développement des colonnes, car l’injection de tetradotoxin (TTX, une molécule qui empêche l’activité synaptique) dans le nerf optique de l’œil greffé empêche la formation des colonnes répondant à ce nouvel organe (Reh & Constantine-Paton, 1985). Le cerveau est donc capable de s’adapter à un nouvel intrant, et de le rendre fonctionnel. Ce fait est d’un grand intérêt pour l’application d’appareils de substitution sensorielle. Le cerveau serait capable de gérer un nouvel œil, mais serait-il capable de gérer un nouveau sens ?
2.3. Études de lésions
Les études citées précédemment avaient pour objectif de tenter de comprendre le rôle des influences environnementales (l’expérience sensorielle) sur le développement des connections corticales. Leur approche était une approche réductive. On diminue ou élimine l’activité de l’organe sensoriel au niveau périphérique (en fermant les paupières ou en énucléant un ou les deux yeux par exemple) pour observer les changements plastiques au niveau central. Une autre approche pour étudier la plasticité du cerveau est d’induire des changements au niveau central et d’étudier l’adaptation du cerveau à ces changements. Ces études peuvent, par exemple, éliminer une aire corticale au complet (ou un centre de relais important comme le CS) pour étudier les changements corticaux qui en découlent.
Schneider (1973) démontra en premier que la lésion de certaines structures sous corticales (le CS) durant la phase prénatale peut engendrer des connections de la rétine vers des zones où elle ne projette pas normalement. De cette façon, on peut créer des connections aberrantes de la rétine vers le cortex somatosensoriel ou auditif (Frost, 1982; Sur, 1988). Ces connections étant fonctionnelles (Frost & Metin, 1985), il y a lieu de se poser la question de savoir si le cortex auditif de ces animaux « re-câblés » peut prendre en charge des tâches visuelles. Chez l’animal normal (Figure 5a), la rétine projette principalement au LGN, et au CS (voie en bleu). Le LGN à son tour, projette principalement au cortex visuel. Le MGN par contre reçoit la majorité des ses afférences du collicule inférieur (CI) ipsilatéral (voie en rouge). Le MGN envoie aussi des projections au cortex visuel. Chez l’animal « re-câblé » (Figure 5b), on détruit le brachuim du CI qui est censé envoyer ses projections au MGN, et le CS aussi est détruit. Dans le cas de ces animaux, les cellules du MGN répondent à des stimuli visuels. En lésant des cibles rétiniennes normales, on peut donc créer des projections permanentes de la rétine vers des cibles non-visuelles, comme le thalamus auditif par exemple (Ptito et al., 2001). Les cellules du cortex somatosensoriel qui reçoivent des projections de la rétine ont des comportements semblables aux cellules du cortex visuel de l’animal normal (Ptito et al., 2001). Les hamsters dont le système visuel est lésé, mais qui possèdent des projections rétiniennes vers le thalamus auditif (corps genouillé médian, ou MGN) ont des activations visuelles de leur cortex auditif (Ptito & Kupers, 2005). De plus, ces cellules adoptent des caractéristiques des cellules du cortex visuel, comme une préférence à l’orientation, à la direction et une sensibilité au mouvement. Du point de vue comportemental, ces animaux « re-câblés » peuvent apprendre des tâches de discrimination visuelle aussi bien que des animaux normaux (Ptito & Kupers, 2005). Ceci indique que le cortex auditif « re-câblé » peut prendre en charge des tâches visuelles, avec la même efficacité que le cortex visuel. En effet, une lésion du cortex auditif de ces animaux aux projections anormales les rend aussi aveugles comme le ferait la lésion du cortex visuel chez le normal. Ceci indique clairement que le cortex auditif de ces animaux a changé d’identité pour prendre en charge les fonctions du cortex lésé. Le rôle perceptif du cortex auditif est changé par ces opérations à un point tel que les animaux « re-câblés » interprètent l’activation du cortex auditif par la stimulation visuelle comme de la vision. Donc, la nature de l’intrant sensoriel à un rôle profond sur la construction des réseaux neuronaux Ce qui est d’une grande importance pour l’application du système de substitution sensorielle. En effet, ces études suggèrent que le cortex pourrait s’accommoder d’un nouvel intrant sensoriel qui substitue la vision, et interpréterait cette information comme de la vision.
3. Études Humaines
3.1. Études de privation sensorielle
Il existe aussi des preuves anatomiques de plasticité intermodale chez l’humain. La plasticité et l’intermodalité chez l’humain peuvent être observées dans des cas de privation sensorielle, de lésions, ou d’apprentissage de nouvelles tâches. Chez l’aveugle, cette plasticité intermodale a des conséquences comportementales importantes. Comme chez le chat aveugle (voir section sur la privation sensorielle chez l’animal) qui performe mieux dans la localisation de stimuli auditifs, on observe chez les aveugles de naissance une compensation tactile et auditive pour l’absence de vision. Déjà en 1749, Diderot abordait le thème de la compensation sensorielle dans son essai : Lettre sur les aveugles. Il rapportait l’habilité avec laquelle certaines personnes aveugles arrivaient à éviter des obstacles et à localiser des sons. William James en 1890 écrivait aussi que la perte d’un sens devrait promouvoir d’extraordinaires habilités perceptuelles des autres sens. Il existe dans l’imaginaire commun l’idée que les individus aveugles ont des capacités perceptuelles compensatrices pour les sens intacts qu’il leur reste. Il existe certaines preuves neuroanatomiques tangibles de cette idée.
Le cortex visuel de sujets aveugles peut être recruté pour accomplir des tâches tactiles ou auditives. Par exemple, le cortex visuel de sujets aveugles est activé par la lecture du braille (Sadato et al., 1996). Dans le modèle de plasticité proposé par Wiesel et Hubel, l’expérience visuelle n’est censée influencer la structuration du cerveau que pendant une période critique. Il a été démontré chez le chat que la fermeture d’une paupière n’avait pas les mêmes conséquences corticales après cette période critique (Rosa, et al, 1995). La plasticité modifie les colonnes de dominance oculaire pendant la période critique seulement.
Pendant longtemps, la littérature dans le domaine de la cécité a entretenu l’idée selon laquelle le cortex visuel sain d’un aveugle devait s’atrophie par manque d’information à cette région corticale. Nous savons aujourd’hui que cette croyance est non fondée. Il est vrai que l’absence d’un œil aura un effet sur les colonnes de dominance oculaire du LGN et que le cortex visuel sera altéré en cas de cécité, mais cette altération est tout le contraire de l’atrophie. Une étude en imagerie révèle que le cortex d’aveugles congénitaux n’est pas seulement actif, mais possède un métabolisme supranormal (De Volder et al., 1997). Cela veut dire que le cortex visuel peut apprendre à intégrer de l’information qui provient d’autres modalités. Le cortex visuel de personnes aveugles de naissance peut être activé par des sons ou de la stimulation tactile, ce qui n’est pas le cas chez les sujets voyants (Sampaio et al., 2000). Le cortex visuel est activé lors de tâches de discrimination tactile (Ptito, et al, 2005), telle la lecture du braille chez les aveugles (Maguire et al., 2000), ou par la stimulation auditive (Kay, 2000) et ceci est très loin de la thèse de l’atrophie. Au contraire, les chercheurs tentent aujourd’hui d’exploiter cette impressionnante capacité d’adaptation du cortex visuel pour rétablir une forme de vision aux aveugles et faciliter la vie de ceux-ci. L’interface tactile ou sonore emploie le même paradigme pour acheminer l’information visuelle au cortex. L’activation du cortex visuel durant la lecture du braille est due à une réorganisation corticale suite à la privation sensorielle. En effet, certaines parties du cortex visuel sont recrutées par d’autres modalités chez les aveugles. La stimulation électrotactile de la langue sollicite aussi le cortex visuel chez les aveugles de naissance dans une tâche de discrimination (Sampaio, et al, 2001). Il a été démontré dans cette étude que le cortex occipital prend en charge la fonction de discrimination tactile somatosensorielle sur la langue. La lettre T est projetée sur la langue sous forme d’influx électriques grâce à une grille de pixels placée sur la langue. Les aveugles ayant appris à discriminer l’orientation de la lettre T ont un taux de succès de 90% ou plus. La stimulation magnétique transcrânienne (TMS) du cortex occipital chez les sujets voyants entraîne l’apparition de phosphènes visuels, tandis que chez les aveugles de naissance dont le cortex visuel est recruté pour des tâches tactile, cette même stimulation entraîne l’apparition de phosphene tactile sur la langue et les doigts (Kupers, et al., 2005). Ceci est une preuve supplémentaire de la réorganisation corticale de ces participants.
La conséquence comportementale de la plasticité corticale est la substitution sensorielle, que ce soit par l’ouïe ou le toucher. La substitution sensorielle est un phénomène naturel, une adaptation du cerveau au changement. La cécité précoce chez l’humain engendre des changements au niveau du cortex par un phénomène de substitution sensorielle. Une grande partie de ce cortex devient auditif ou tactile. De la même façon que le cortex visuel de sujets aveugles s’adapte à la cécité, le cortex auditif de personnes sourdes-nées est plastique et peut adapter de l’information provenant d’autres sens. Les sourds qui apprennent à lire sur les lèvres ont un cortex auditif qui répond au visionnement du mouvement des lèvres (Crane & Milner, 2005).
La question qui se pose maintenant est de savoir s’il existe une période critique pour ces changements, comme il a été démontré pour les colonnes de dominance oculaire chez le chat. Il semblerait que les personnes aveugles, sans égard face à l’âge de la cécité, ont une plus grande activation du cortex visuel associatif que les voyants dans des tâches de discriminations tactiles. Par contre, l’activation du cortex visuel proprement dit (V1) n’était activé que chez les sujets dont la cécité était apparue avant l’âge de 16 ans (Sadato, et al., 2002). Il semblerait donc improbable que V1 soit la porte d’entrée pour la redirection des signaux tactiles vers le cortex visuel. Par contre, le cortex visuel associatif pourrait moduler la circuiterie grâce à laquelle V1 est activé lors de tâches de discrimination tactile. Le cortex visuel associatif est activé chez des personnes de cécité tardive sans aucun entraînement au braille, mais pas chez les voyants (Sadato et al., 2004). Ceci implique que la plasticité corticale au niveau du cortex associatif est possible même au-delà de la période critique. Les aveugles de naissance qui apprennent à faire des discriminations tactiles avec la langue n’ont pas d’activation de V1 avant l’entraînement, mais suite à un entraînement, V1 est activé (Ptito et al., 2005). Ceci peut vouloir dire que, suite à un entraînement, le cortex visuel associatif peut rediriger l’intrant tactile vers V1. Une étude de localisation de sons dans l’espace chez les aveugles de cécité tardive démontre une réorganisation du cortex occipital. Cette tâche active le cortex occipital des sujets de cécité tardive, aussi bien que ceux de cécité précoce
Figure 6:
La partie postérieur de l’hippocampe est significativement réduite (p<.05) astérisk) chez les aveugles (bleu) comparé au voyants (rouge). Chebat et al., 2006, OHBM.(Voss, et al., 2006). 3.2. Études additives Un autre exemple de plasticité du cerveau suite à un entraînement a été démontré chez les chauffeurs de taxi. Les chauffeurs de taxi de Londres au Royaume-Uni subissent un entraînement intensif et doivent connaître les rues de cette ville presque par cœur. Suite à cet entraînement, on observe une augmentation de la partie postérieure de l’hippocampe (Maguire et al., 2000). L’hippocampe est une zone de mémoire, et la partie postérieure est impliquée dans la navigation (O'Keefe & Speakman, 1987; O'Keefe, 1991). Ces changements structuraux adaptatifs ont eu lieu longtemps après la période critique, puisque leur entraînement se fait à l’âge adulte (leur entraînement se faisant dans l’âge adulte seulement). Cette zone est donc modifiable durant un laps de temps qui va au-delà de la période critique. Cette zone est réduite chez les personnes aveugles de naissance (Chebat et al., 2006b). Plusieurs études ont démontré l’implication de l’hippocampe dans la mémoire topographique des lieux (pour une revue voir: (Maguire, 1997) et des représentations allocentriques de l’espace (Holdstock et al., 2000; O'Keefe, 1991). La zone antérieure de l’insula/ventrolatéral du cortex préfrontal et paritétal est aussi activée à plusieurs moments du processus de navigation (Spiers & Maguire, 2006). D’autres études ont démontré une modulation de l’interaction entre l’hippocampe et les régions frontales et pariétales du cortex dépendamment du type de stratégie employé dans la navigation (Mellet et al., 2000). Il semblerait que les régions médio-pariétales jouent un rôle dans l’analyse du mouvement dans l’espace immédiat et que les régions pariétales jouent un rôle dans la planification du mouvement dans l’espace qui n’est pas accessible visuellement (Spiers & Maguire, 2006). Il serait donc possible que les sujets aveugles aient subi des changements plastiques dans leur système de navigation pour compenser l’absence de vision avec la contribution de régions pariétales et médianes pour analyser et se mouvoir dans l’espace. 3.3. Études de lésions Une autre façon d’observer des changements de fonction dans le cerveau est l’étude de sujets cérébrolésés. Les causes de lésions corticales peuvent être multiples, un accident causant un traumatisme crânien par exemple. L’épilepsie peut aussi entraîner la mort cellulaire et parfois même d’un hémisphère au complet. On traite souvent l’épilepsie dans ses cas les plus graves par des callosotomies (sectionnement du corps calleux) ou une hémisphèrectomie. Une hémisphèrectomie consiste à retirer au complet l’hémisphère malade pour traiter les crises épileptiques. Ce genre d’opération peut engendrer des conséquences comportementales très graves. L’absence d’un hémisphère cause habituellement la cécité dans l’hémichamp controlatéral. L’hémisphère ayant été supprimé, il n’y a plus de cortex visuel pour analyser l’information. Pourtant, la présentation d’un stimulus dans l’hémichamp qui est censé être aveugle a une influence sur la réaction au stimulus présenté dans l’hémichamp sain. Bien qu’aucun des patients n’était conscient des stimuli dans l’hémichamp aveugle, leur temps de réaction était plus court quand ils étaient présentés simultanément (Tomaiuolo, et al , 1997). Ceci est surprenant car la présentation de deux stimuli simultanés facilite toujours le temps de réaction par rapport à deux stimuli discordants chez un sujet normal. Une analyse d’imagerie fonctionnelle sur ces patients révèle que la présentation d’un stimulus dans l’hémichamp aveugle active chez les sujets lésés le cortex visuel ipsilatéral à la lésion (Bittar et al., 1999). Or, il s’avère que chez le sujet normal, cette stimulation active toujours le cortex controlatéral. Ceci suggère que le cortex ipsilatéral a subi des modifications plastiques pour accommoder l’hémichamp aveugle. Le rétablissement spontané des fonctions perdues à cause de la lésion ne se voit que dans des cas d’anomalies congénitales (Ptito, et al, 1999). Par contre, beaucoup de personnes ayant subi des lésions plus tard dans la vie exhibent ce qu’on appelle le Blind Sight. Le blind sight est un phénomène où l’information de l’hémichamp aveugle a une influence sur le traitement de l’information de l’hémichamp sain. Dans les cas de blind sight, les sujets ayant une cécité corticale arrivent à reconnaître des stimuli présentés dans leur hémichamp aveugle dans le cadre d’un choix forcé (Weiskrantz, et al., 1974). Ils ne sont pas conscients de l’information qui leur est présentée, mais cette information est utilisée par le cerveau. Les patients hémisphèrectomisés perdent d’abord l’usage de certaines fonctions motrices qui étaient contrôlées par l’hémichamp supprimé, et ont une réduction de leurs champ visuel à cause de l’absence de cet hémisphère. Pourtant, après un certain temps, on observe une amélioration des fonctions motrices perdues (Olausson et al., 2001), et de leur champ visuel (Fendrich, et al., 2001 ; Ptito et al., 2001). Il semblerait donc que la quasi-totalité des fonctions perdues seraient transférée vers l’hémisphère controlatéral, ce qui implique une réorganisation massive du cerveau. Ces observations soulèvent la question suivante: par où l’information de la rétine passe-t-elle pour atteindre le cortex ipsilatéral ? Le LGN ipsilatéral à l’hémisphère aboli subi une dégénération massive (Boire, et al., 2000), il semblerait donc improbable que l’information rétinienne emploie cette voie pour atteindre l’hémisphère intact. Par contre, le CS subi seulement une faible réduction de volume, et on retrouve des projections rétiniennes dans le CS ipsilatéral à la lésion, ce qui suggère que cette structure maintient ces fonctions visuelles (Boire, et al., 2001). Le CS est une structure visuelle sous corticale, dite inconsciente car elle gère la transformation d’information visuelle en reflexes moteurs. Cette structure est donc bien placée pour transférer l’information visuelle résiduelle via sa commissure vers le cortex visuel ipsilatéral. Le rôle de la commissure du collicule supérieure (CCS) dans le blind sight est confirmé par une étude récente en neuroimagerie (Leh, et al., 2006). La CCS pourrait donc prendre en charge certaines fonctions résiduelles du cortex lésé dans le blind sight, et serait donc un instrument indispensable dans ce mécanisme de plasticité. Conclusion Nous avons montré dans ce texte de synthèse le rôle important de l’expérience dans le modelage des connexions corticales. Sans l’apport de l’expérience visuelle et de l’activité neuronale pour stimuler et altérer la force des connexions synaptiques, le cerveau ne se développera pas normalement. L’expérience sensorielle par le biais de mécanismes de plasticité continue d’influencer le cerveau durant toute son existence. Nous avons démontré ce fait par plusieurs études : les études de privation sensorielle, d’apprentissage de nouvelle tâche et de rétablissement en cas de lésions. Bien que le cerveau démontre moins de plasticité dans l’âge adulte, les colonnes de dominance oculaire n’étant modifiables que durant un laps de temps précis, d’autres aspects du cerveau sont modifiables durant toute son existence, comme le démontrent les études sur la cécité et l’apprentissage chez l’humain. Certaines études démontrent que l’activation du cortex visuel est possible même chez des personnes atteintes de cécité tard dans la vie. Les études de modification structurelle de l’hippocampe chez les chauffeurs de taxi sont un autre exemple de plasticité tardive. Le cerveau est capable de s’adapter aux besoins changeants de notre environnement. Le cerveau peut s’accommoder des différents intrants sensoriels, comme l’ajout d’une aire corticale ou d’un troisième œil. Dans mon texte de projet de recherche intitulé « Aspects neuro-cognitifs du processus de la navigation chez l’aveugle-né », j’ai décrit les différents appareils de substitution sensorielle qui tentent d’exploiter les mécanismes de plasticité décrits dans le présent texte. À la lumière des changements profonds induits par l’expérience sensorielle durant le développement, il est possible de croire que le cerveau pourrait intégrer l’information fournie par ces appareils comme de la vision subjective.
Berardi, N., Pizzorusso, T., Ratto, G. M., & Maffei, L. (2003). Molecular basis of plasticity in the visual cortex. Trends Neurosci, 26(7), 369-378.
Bittar, R.G., Olivier, A., Sadikot, A.F., Anderman, F., Pike, G.B., Reutens, D.C. (1999) Presurgical motor and somatosensory cortex mapping with functional magnetic resonance imagery and positron emission tomography. J Neursurg, 91(6)915-921
Blakemore, C., Van Sluyters, C. V., & Movshon, J. A. (1976). Synaptic competition in the kitten's visual cortex. Cold Spring Harb Symp Quant Biol, 40, 601-609.
Boire, D., Dufour, J. S., Theoret, H., & Ptito, M. (2001). Quantitative analysis of the retinal ganglion cell layer in the ostrich, Struthio camelus. Brain Behav Evol, 58(6), 343-355.
Boire, D., Theoret, H., Herbin, M., Casanova, C., & Ptito, M. (2000) Retinogeniculate projections following early cerebral hemispherectomy in the vervet monkey. Exp Brain Res, 135(3): 373-381.
Bronchti, G., Heil, P., Sadka, R., Hess, A., Scheich, H., & Wollberg, Z. (2002). Auditory activation of "visual" cortical areas in the blind mole rat (Spalax ehrenbergi). Eur J Neurosci, 16(2), 311-329.
Chebat, D. R., Boire, D., & Ptito, M. (2006)a. Development of the commissure of the superior colliculus in the hamster. J Comp Neurol, 494(6), 887-902.t
Chebat, D.R., Chen, J.K., Ptito., A., Schneider, F., Kupers, R., Ptito, M. (2006) Volumetric anlysis of the hippocmapus in early nlind subjects. OHBM , Florence, Italy.
Constantine-Paton, M., & Law, M. I. (1978). Eye-specific termination bands in tecta of three-eyed frogs. Science, 202(4368), 639-641.
Crane, J., & Milner, B. (2005). What went where? Impaired object-location learning in patients with right hippocampal lesions. Hippocampus, 15(2), 216-231.
Crowley, J. C., & Katz, L. C. (1999). Development of ocular dominance columns in the absence of retinal input. Nat Neurosci, 2(12), 1125-1130.
Crowley, J. C., & Katz, L. C. (2000). Early development of ocular dominance columns. Science, 290(5495), 1321-1324.
De Volder, A. G., Bol, A., Blin, J., Robert, A., Arno, P., Grandin, C., Michel, C., & Veraart, C. (1997). Brain energy metabolism in early blind subjects: neural activity in the visual cortex. Brain Res, 750(1-2), 235-244.
Dehay, C., Giroud, P., Berland, M., Killackey, H., & Kennedy, H. (1996a). Contribution of thalamic input to the specification of cytoarchitectonic cortical fields in the primate: effects of bilateral enucleation in the fetal monkey on the boundaries, dimensions, and gyrification of striate and extrastriate cortex. J Comp Neurol, 367(1), 70-89.
Dehay, C., Giroud, P., Berland, M., Killackey, H. P., & Kennedy, H. (1996b). Phenotypic characterisation of respecified visual cortex subsequent to prenatal enucleation in the monkey: development of acetylcholinesterase and cytochrome oxidase patterns. J Comp Neurol, 376(3), 386-402.
Feldman, D. E., & Brecht, M. (2005). Map plasticity in somatosensory cortex. Science, 310(5749), 810-815.
Fendrich, R., Wessinger, C.M., & Gazzaniga, M.S. (2001) Speculations on the neural basis of islands of blindsight. Prog Brain Res, 134: 353-366
Frost, D. O. (1982). Anomalous visual connections to somatosensory and auditory systems following brain lesions in early life. Brain Res, 255(4), 627-635.
Frost, D. O., Boire, D., Gingras, G., & Ptito, M. (2000). Surgically created neural pathways mediate visual pattern discrimination. Proc Natl Acad Sci U S A, 97(20), 11068-11073.
Frost, D. O., & Metin, C. (1985). Induction of functional retinal projections to the somatosensory system. Nature, 317(6033), 162-164.
Guillery, R. W., & Stelzner, D. J. (1970). The differential effects of unilateral lid closure upon the monocular and binocular segments of the dorsal lateral geniculate nucleus in the cat. J Comp Neurol, 139(4), 413-421.
HEBB, C. O., & KONZETT, H. (1949). The effect of certain analgesic drugs on synaptic transmission as observed in the perfused superior cervical ganglion of the cat. Q J Exp Physiol Cogn Med Sci, 35(3), 213-217.
Holdstock, J. S., Mayes, A. R., Cezayirli, E., Isaac, C. L., Aggleton, J. P., & Roberts, N. (2000). A comparison of egocentric and allocentric spatial memory in a patient with selective hippocampal damage. Neuropsychologia, 38(4), 410-425.
Innocenti, G. M. (1978). Postnatal development of interhemispheric connections of the cat visual cortex. Arch Ital Biol, 116(3-4), 463-470.
Innocenti, G. M., & Frost, D. O. (1980). The postnatal development of visual callosal connections in the absence of visual experience or of the eyes. Exp Brain Res, 39(4), 365-375.
Kandel, E. R. (2001). The molecular biology of memory storage: a dialogue between genes and synapses. Science, 294(5544), 1030-1038.
Korte, M., & Rauschecker, J. P. (1993). Auditory spatial tuning of cortical neurons is sharpened in cats with early blindness. J Neurophysiol, 70(4), 1717-1721.
Kupers, R., Fumal, A., Schonen, F., & Ptito, M., (2005) Tactile phosphene elicited by TMS in the blind: new evidence for the development of cross modal plasticity. OHBM 2005, Toronto, Canada.
Law, M. I., & Constantine-Paton, M. (1980). Right and left eye bands in frogs with unilateral tectal ablations. Proc Natl Acad Sci U S A, 77(4), 2314-2318.
Law, M. I., & Constantine-Paton, M. (1981). Anatomy and physiology of experimentally produced striped tecta. J Neurosci, 1(7), 741-759.
Leh, S. E., Johansen-Berg, H., & Ptito, A. (2006). Unconscious vision: new insights into the neuronal correlate of blindsight using diffusion tractography. Brain, 129(Pt 7), 1822-1832.
Maguire, E. A. (1997). Hippocampal involvement in human topographical memory: evidence from functional imaging. Philos Trans R Soc Lond B Biol Sci, 352(1360), 1475-80.
Maguire, E. A., Gadian, D. G., Johnsrude, I. S., Good, C. D., Ashburner, J., Frackowiak, R. S., & Frith, C. D. (2000). Navigation-related structural change in the hippocampi of taxi drivers. Proc Natl Acad Sci U S A, 97(8), 4398-403.
Maguire, E. A., Mummery, C. J., & Buchel, C. (2000). Patterns of hippocampal-cortical interaction dissociate temporal lobe memory subsystems. Hippocampus, 10(4), 475-82.
Mellet, E., Briscogne, S., Tzourio-Mazoyer, N., Ghaem, O., Petit, L., Zago, L., Etard, O., Berthoz, A., Mazoyer, B., & Denis, M. (2000). Neural correlates of topographic mental exploration: the impact of route versus survey perspective learning. Neuroimage, 12(5), 588-600.
Miller, B., Windrem, M. S., & Finlay, B. L. (1991). Thalamic ablations and neocortical development: alterations in thalamic and callosal connectivity. Cereb Cortex, 1(3), 241-61.
O'Keefe, J. (1991). An allocentric spatial model for the hippocampal cognitive map. Hippocampus, 1(3), 230-5.
O'Keefe, J., & Speakman, A. (1987). Single unit activity in the rat hippocampus during a spatial memory task. Exp Brain Res, 68(1), 1-27.
Olausson, H., Marchand, S., Bittar, R.G., Bernier, J., Ptito, A., Bushnell, M.C. (2001) Central Pain in a hemispherctomized patient. Eur J Pain, 5(2) : 209-217
Olavarria, J. F., & Hiroi, R. (2003). Retinal influences specify cortico-cortical maps by postnatal day six in rats and mice. J Comp Neurol, 459(2), 156-72.
Ptito, A., Fortin, A., & Ptito, M. (2001) `Seeing`in the blind hemifield following hemispherectomy. Prog Brain Res, 134: 367-378
Ptito, M., Bouchard, P., Lepore, F., Quessy, S., Di Stefano, M., & Guillemot, J. P. (1995). Binocular interactions and visual acuity loss in esotropic cats. Can J Physiol Pharmacol, 73(9), 1398-405.
Ptito, M., Dalby, M., Gjedde, A., (1999) Visual field recovery in a patient with bilateral occipital lobe damage. Acta Neurol Scand, 99(4): 252-254.
Ptito, M., Desgent, S., Sensory input-based adaptation and brain architecture (2006) Lifespan development and the brain. Baltes, New-York press.
Ptito, M., Giguere, J. F., Boire, D., Frost, D. O., & Casanova, C. (2001). When the auditory cortex turns visual. Prog Brain Res, 134, 447-58.
Ptito, M., & Kupers, R. (2005). Cross-modal plasticity in early blindness. J Integr Neurosci, 4(4), 479-88.
Ptito, M., Moesgaard, S. M., Gjedde, A., & Kupers, R. (2005). Cross-modal plasticity revealed by electrotactile stimulation of the tongue in the congenitally blind. Brain, 128(Pt 3), 606-14.
Rakic, P., Suner, I., & Williams, R. W. (1991). A novel cytoarchitectonic area induced experimentally within the primate visual cortex. Proc Natl Acad Sci U S A, 88(6), 2083-7.
Rampon, C., & Tsien, J. Z. (2000). Genetic analysis of learning behavior-induced structural plasticity. Hippocampus, 10(5), 605-9.
Rauschecker, J. P., & Kniepert, U. (1994). Auditory localization behaviour in visually deprived cats. Eur J Neurosci, 6(1), 149-60.
Rauschecker, J. P., & Korte, M. (1993). Auditory compensation for early blindness in cat cerebral cortex. J Neurosci, 13(10), 4538-48.
Rebillard, G., Carlier, E., & Pujol, R. (1977). [Visual evoked responses on the primary auditory cortex in the cat after an early suppression of cochlear receptors (author's transl)]. Rev Electroencephalogr Neurophysiol Clin, 7(3), 284-9.
Rebillard, G., Carlier, E., Rebillard, M., & Pujol, R. (1977). Enhancement of visual responses on the primary auditory cortex of the cat after an early destruction of cochlear receptors. Brain Res, 129(1), 162-4.
Reh, T. A., & Constantine-Paton, M. (1985). Eye-specific segregation requires neural activity in three-eyed Rana pipiens. J Neurosci, 5(5), 1132-43.
Rhoades, R. W., & Fish, S. E. (1982). Altered organization of intercollicular pathway in bilaterally enucleated hamsters. Brain Res, 256(3), 356-60.
Rosa, M. G., Schmid, L. M., & Calford, M. B. (1995). Responsiveness of cat area 17 after monocular inactivation: limitation of topographic plasticity in adult cortex. J Physiol, 482 ( Pt 3), 589-608.
Rubenstein, J. L., Anderson, S., Shi, L., Miyashita-Lin, E., Bulfone, A., & Hevner, R. (1999). Genetic control of cortical regionalization and connectivity. Cereb Cortex, 9(6), 524-32.
Sadato, N., Okada, T., Honda, M., & Yonekura, Y. (2002). Critical period for cross-modal plasticity in blind humans: a functional MRI study. Neuroimage, 16(2), 389-400.
Sadato, N., Okada, T., Kubota, K., & Yonekura, Y. (2004). Tactile discrimination activates the visual cortex of the recently blind naive to Braille: a functional magnetic resonance imaging study in humans. Neurosci Lett, 359(1-2), 49-52.
Sadato, N., Pascual-Leone, A., Grafman, J., Ibanez, V., Deiber, M. P., Dold, G., & Hallett, M. (1996). Activation of the primary visual cortex by Braille reading in blind subjects. Nature, 380(6574), 526-8.
Sampaio, E. (1994). [The early development of blind children: the pioneering work of Selma Fraiberg]. Psychiatr Enfant, 37(1), 29-47.
Sampaio, E., Maris, S., & Bach-y-Rita, P. (2001). Brain plasticity: 'visual' acuity of blind persons via the tongue. Brain Res, 908(2), 204-7.
Sampaio, E., & Philip, J. (1995). Influences of age at onset of blindness on Braille reading performances with left and right hands. Percept Mot Skills, 81(1), 131-41.
Schneider, G. E. (1973). Early lesions of superior colliculus: factors affecting the formation of abnormal retinal projections. Brain Behav Evol, 8(1), 73-109.
Sengpiel, F., Stawinski, P., & Bonhoeffer, T. (1999). Influence of experience on orientation maps in cat visual cortex. Nat Neurosci, 2(8), 727-32.
Sincich, L. C., & Horton, J. C. (2005). Input to V2 thin stripes arises from V1 cytochrome oxidase patches. J Neurosci, 25(44), 10087-93.
Spiers, H. J., & Maguire, E. A. (2006). Thoughts, behaviour, and brain dynamics during navigation in the real world. Neuroimage.
Sur, M. (1988). Visual projections induced into auditory thalamus and cortex: implications for thalamic and cortical information processing. Prog Brain Res, 75, 129-36.
Sur, M., Garraghty, P. E., & Roe, A. W. (1988). Experimentally induced visual projections into auditory thalamus and cortex. Science, 242(4884), 1437-41.
Sur, M., & Leamey, C. A. (2001). Development and plasticity of cortical areas and networks. Nat Rev Neurosci, 2(4), 251-62.
Sur, M., & Rubenstein, J. L. (2005). Patterning and plasticity of the cerebral cortex. Science, 310(5749), 805-10.
Toldi, J., Farkas, T., & Volgyi, B. (1994). Neonatal enucleation induces cross-modal changes in the barrel cortex of rat. A behavioural and electrophysiological study. Neurosci Lett, 167(1-2), 1-4.
Toldi, J., Rojik, I., & Feher, O. (1994). Neonatal monocular enucleation-induced cross-modal effects observed in the cortex of adult rat. Neuroscience, 62(1), 105-14.
Tomaiuolo, F., Ptito, M., Marzi, C.A., Paus, T., Ptito, A., (1997)Blindsight in hemespherectomised patients as revealed by spatial summation across the vertical meridian. Brain,120(5), 795-803
Voss, P., Gougoux, F., Lassonde, M., Zatorre, R. J., & Lepore, F. (2006). A positron emission tomography study during auditory localization by late-onset blind individuals. Neuroreport, 17(4), 383-8.
Wiesel, T. N., & Hubel, D. H. (1965). Comparison of the effects of unilateral and bilateral eye closure on cortical unit responses in kittens. J Neurophysiol, 28(6), 1029-40.
Weiskrantz, L., Warrington, E.K., Sanders, M.D., & Marshall, J. (1974) Visual capacity in the hemianopic field following a restrcited occipital ablation. Brain 97(4): 709-728.
Windrem, M. S., & Finlay, B. L. (1991). Thalamic ablations and neocortical development: alterations of cortical cytoarchitecture and cell number. Cereb Cortex, 1(3), 230-40.
Yu, H., Farley, B. J., Jin, D. Z., & Sur, M. (2005). The coordinated mapping of visual space and response features in visual cortex. Neuron, 47(2), 267-80.
Saturday, June 17, 2006
Human Brain Mapping Convention 2006, Florence Italy.
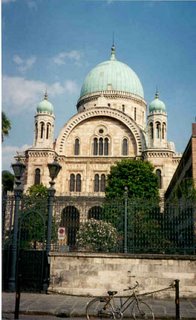

I have just returned from the 2006 OHBM convention in Florence, Italy. It was my first OHBM convention, and also my first time in Italy. I really enjoyed this convention and presenting my results there (see: http://www.humanbrainmapping.org/, or you can view my abstract in an earlier entry of this weblog).
Saturday, June 10, 2006
What is the Tongue Display Unit (TDU)?

The TDU is a device that can help transmit visual information via the tongue using electro-tactile signals. The dream of beiong able to restore certain functions of vision via tactile senses is actually a very old one, but one we are only beginning to approach today thanks, in part, to a better understanding of how the brain functions, and also to recent technological adavancements like the miniaturisation of computers and capture devices like web-cams.
The first visual-tactile interface system was developed by Dr. Paul Bach-y-Rita in the 1970's. The original tactile visual sensory substitution device (TVSS) was very large (due in part to the large volume of computers and cameras then) and it stimulated the tactile surface of the back to transmit visual information.
This system was later adapted by scientist (Kurt Kaczmarek, and Paul Bach-y-Rita) at the University of Wisconsin to use the tactile sense of the tongue to transmit the visual information to the brain. For more information please see: http://kaz.med.wisc.edu/Publicity/Synopsis.html
This system was given to the Maurice Ptito laboratory in vision research in 2005 to help develop the system and our understanding of brain plasticity and sensory Substitution. Basically the system that we have is a webacm that is connected to a laptop. The laptop communicates with the tongue device through a wireless bluetooth connection that sends the image that is being capted by the camera in real time to the tongue stimulator aray. This aray consists of a grid of electrodes that can recreate the image with electricity on the tongue. I had the honour of meeting Dr. Paul Bach-y-Rita on the inauguration of the Harland Sanders Chair in visual science. We had very interesting conversations on the nature of sensory substitution and the philosophical implications of brain plasticity. My goal as a reasearcher is to find out if it is possible to use this device for navigation and also what cortical regions will be elicited in this task.
Volumetric analysis of the hippocampus in early blind subjects


1Chaire de recherche Harland Sanders, Ecole d’Optométrie, Université de Montréal, Qc, Canada; 2Neuropsychology, Montreal Neurological Institute, McGill University, Qc, Canada; 3Pet center, Riggs Hospital, Copenhagen, Denmark; 4Danish Research Centre for MR, Copenhagen University Hospital, Hvidovre, Copenhagen, Denmark.
Welcome to my blog!

D-R. Chebat, D. Boire, M. Ptito (2006) Development of the commissure of the superior colliculus in the hamster. J Comp Neurol. Feb 20;494(6):887-902
D-R. Chebat, J-K. Chen, A. Ptito, R. Kupers, F. Schneider, M. Ptito (2006) Volumetric analysis of the hippocampus in early blind subjects Human Brain Mapping, Florence Italy
Wednesday, June 07, 2006
La négociation d’obstacle par des aveugles de naissance à l’aide de stimulation linguale électro-tactile
Nous étudions les capacités de personnes aveugles nés , de cécité tardive, et voyante dans la perception et la négociation d'obstacles dans des tâches de navigation et d’orientation dans l’espace a l’aide de l’appareil de stimulation linguale (Tongue Display Unit- TDU). Une étude de cas démontre décris les perceptions d’un sujet utilisant le TVSS pour identifier et localiser certains objets (Guarniero, 1974). Ces études et les avancées technologique permettent de croire qu’il serait possible pour un sujet aveugle de naissance d’apprendre à décoder l’information capté par ces appareils pour se déplacer et éviter des obstacles dans un environnement qui lui est inconnu. Aucune étude n’a étudié les capacités des aveugles de s’orienter dans un parcours à obstacle utilisant un appareil de substitution sensorielle, tel le Tongue Display Unit (TDU).